18 April 2024
PLEASE NOTE: The Viewpoints on our website are to be read and freely shared by all. If they are republished, the following text should be used: “This Viewpoint was originally published on the REVIVE website revive.gardp.org, an activity of the Global Antibiotic Research & Development Partnership (GARDP).”
The views and opinions expressed in this article are solely those of the original author(s) and do not necessarily represent those of GARDP, their donors and partners, or other collaborators and contributors. GARDP is not responsible for the content of external sites.
A major roadblock in developing new antibiotics effective against Gram-negative bacteria is overcoming the permeability barrier. Furthermore, there is a lack of understanding of how discovered hit compounds should be modified to increase their intracellular accumulation and achieve effective intracellular concentrations. Permeability barriers of Gram-negative bacteria vary in their lipid and protein compositions, but all comprise two membranes with poly-specific efflux pumps. Efflux pumps and the permeability barriers of the two membranes act synergistically and often reduce the efficacy of antibiotics by several orders of magnitude.1 Recent studies showed that a successful lead optimization needs to consider both the outer membrane (OM) and the active efflux barrier. Although there are some common themes in current strategies for enhancing the permeability of the outer membrane, the final approaches are typically species- and compound-specific.
Permeation through porins – mimicking the properties of substrates
This approach is the oldest and most well-validated but can be applied to relatively small water-soluble molecules (up to an exclusion size of ≈600 kDa for Escherichia coli). The permeation through E. coli classical porins is well characterized and well described with a scoring function.2 The generalized “rules” of permeation through enterobacterial porins emphasize the size of the molecule and electrostatic interactions of the diffusing molecule with the porin through its charge and dipole. These properties are also identified as top descriptors of antibacterial activities of beta-lactams and fluoroquinolones in E. coli3 and intracellular accumulation experiments.4 Applying “rules” of permeation during lead optimization has yielded several compounds that gained potency against enterobacteria.4 These rules, however, do not apply to Pseudomonas aeruginosa, which, in contrast to E. coli, lack classical porins and relies on substrate-specific porins with a smaller inner diameter for nutrient uptake. One of these porins is OprD, a basic amino acid-specific porin.5 Carbapenems that structurally resemble basic amino acids use OprD to cross the outer membrane of P. aeruginosa.6 Optimization of permeation through multiple porins has yielded good anti-pseudomonal activities for novel inhibitors of penicillin-binding proteins; these inhibitors are small hydrophilic molecules structurally related to beta-lactamase inhibitors.7
A better understanding of efflux inhibition mechanisms and enhancing inhibitory properties in antibacterial compounds could lead to powerful combination therapies with high clinical efficacy, low resistance liability and low toxicities.
Like P. aeruginosa, Acinetobacter baumannii also lacks classical porins and its OM permeability properties are distinct from those of E. coli and P. aeruginosa.8,9 One of the reasons for the differences is the structure of the A. baumannii lipopolysaccharides, which are typically hepta-acylated.10 This extra acyl chain improves the packing of lipid A in the OM, further reducing permeation of the charged and hydrophilic molecules but making the OM more permeable for amphiphilic compounds.11 Among A. baumannii nutrient uptake Occ porins, OccAB1 has the largest pore and could potentially be targeted for optimization of antibiotic uptake.12
Porin-independent pathway – what is it?
Porin-independent pathways are highly diverse, antibiotic-specific, and mostly poorly defined. The exploitation of active uptake across the OM yielded cefiderocol, the first clinically approved antibiotic actively taken into the cells by siderophore transporters.13 However, some antibiotics can use the OM ligand-gated porins to gain intracellular uptake. For example, the rifampicin analogue rifabutin is actively transported across the OM of A. baumannii by a siderophore-gated porin FhuE14. In another example, the P. aeruginosa siderophore-gated porin FoxA interacts with and transports both the thiopeptide antibiotic thiocillin and xenosiderophore nocardamine.15 It is likely that the list of non-conjugated antibiotics that penetrate the OM through the uptake transporters of siderophores, vitamins, peptides, oligosaccharides, etc., is much longer. More systematic studies are needed to understand what properties and molecular interactions enable this mechanism of OM penetration.
The penetration of nutrients across the OM is primarily unidirectional because they are captured with high affinities by periplasmic substrate-binding proteins (PSBPs) and can be accumulated inside the cells in excess of up to 106 fold.16 Practically all of the bacterial uptake of small molecules occurs together with the binding proteins, which are either anchored via a lipid moiety to the cytoplasmic membrane in Gram-positive bacteria or located in the periplasm of Gram-negative bacteria and are bound to respective transporters.17,18 PSBPs confer a high affinity to the transport process with typical KM (the affinity of an enzyme for a given substrate) values around 0.01-1μM but still can handle diverse substrates. For example, the inner membrane oligopeptide transport system (Opp) can handle peptides from two to five amino acid residues composed of a large variety of natural and/or modified residues.19 Such promiscuity comes with a price: Opp systems are implicated in the uptake of various peptidic antibiotics and antimicrobial peptides, such as pacidamycin, an antibiotic with activity against antibiotic-resistant P. aeruginosa.20,21 More recently a PSBP of a purine nucleoside transporter from Borreliella burgdorferi was implicated in active uptake of hygromycin A and was responsible for the species-specific antibacterial activity of this antibiotic.22
Inhibition of efflux pumps such as MexXY-OprM in P. aeruginosa may significantly increase the therapeutic index of many antibiotics, including aminoglycosides, and improve their clinical utility.
A large number of antibiotics are glycosides.23 Natural aminoglycosides and macrolides have been widely prescribed for the treatment of bacterial infections, with gentamicin being one of the most used to treat infections by Gram-negative bacteria. In the early years of antibiotics, the carbohydrate moieties in glycosidic antibiotics were mainly believed to modify their pharmacokinetic parameters, such as solubility, transport, and bioavailability, and modulate their toxicity. Surprisingly, little is known about how certain sugars enhance the permeation of antibiotics across the OM and how large oligosaccharides, such as aminoglycosides, are transported across the inner membrane (IM).
As expected for such large antibiotics as macrolides, the OM is the major barrier for their penetration in both E. coli and P. aeruginosa.8 However, solithromycin (MW, 845 Da) was the only macrolide with moderate activity against wild-type P. aeruginosa (MIC, 12.5 μM), suggesting that this antibiotic utilizes an uptake pathway inaccessible to other macrolides. Furthermore, macrolides also varied in their permeation across the OM of E. coli. Some macrolides were more strongly impeded by the OM barrier than others, suggesting that these antibiotics might utilize several different uptake pathways. A linear correlation in the penetration of other antibiotics, such as fluoroquinolones and sulfonamides into E. coli and P. aeruginosa cells further points to the important role of porin-independent pathways in their permeation as well.8
Permeability enhancers: outer membrane disruptors and efflux pump inhibitors
Polycationic antibiotics such as polymyxins and aminoglycosides penetrate the OM of most Gram-negative bacteria by directly binding to lipopolysaccharides (LPS) and displacing divalent magnesium and calcium cations from the outer leaflet of the OM. This mechanism of a self-promoted uptake was initially proposed by Robert Hancock for aminoglycosides24 and recently revealed at the molecular level for polymyxins.25 The ability of polymyxins and other polycationic compounds to disrupt the integrity of the OM suggested a viable strategy of using such compounds in combinations with other antibiotics for enhancing their OM penetration and antibacterial activities.26
In a recent analysis,27 properties associated with the self-promoted uptake, such as positive charge, hydrogen-bond donors and positive polar surface area of the compound, were identified as the only features predictive of the intracellular accumulation of small molecule compounds in P. aeruginosa. Whether or not all cationic antibiotics penetrate these cells by disrupting the integrity of the OM remains unclear. One of the common behaviors of cationic compounds interacting with the OM is their antagonism with divalent cations, which easily outcompete antibiotics for binding to LPS. At least in the case of aminoglycosides, the famous antagonism of their antibacterial activities with divalent cations in P. aeruginosa requires the function of the multidrug efflux pump MexXY-OprM.28 Hence, inhibition of this efflux pump should abolish the antagonism of aminoglycosides by divalent cations, regardless of its precise mechanism.
Efflux pumps and the permeability barriers of the two membranes act synergistically and often reduce the efficacy of antibiotics by several orders of magnitude.
Despite all the above, the OM is a passive permeability barrier and is rendered less effective in the absence of active drug efflux. Inhibition of efflux pumps such as MexXY-OprM in P. aeruginosa may significantly increase the therapeutic index of many antibiotics, including aminoglycosides, and improve their clinical utility.29,30 Furthermore, several in vitro studies have provided strong evidence that, in the absence of efflux, the emergence of unrelated resistance mechanisms might be significantly suppressed.31,32,33 This phenomenon is seen not only for approved antibiotics but also for compounds at various stages of pre-clinical research30. The underlying molecular mechanisms of such resistance ‘suppression’ remain unclear but the diverse roles of efflux pumps in various aspects of bacterial physiology and virulence may be involved. Interestingly, many substrates of efflux pumps are also their inhibitors when combined with antibiotics. Still, the two classes of ligands apparently utilize different translocation paths within the transporter.34,35 A better understanding of efflux inhibition mechanisms and enhancing inhibitory properties in antibacterial compounds could lead to powerful combination therapies with high clinical efficacy, low resistance liability and low toxicities.
Permeability barriers of Gram-negative bacteria have been optimized by eons of evolution to protect cells from exogeneous threats while maximizing the uptake of nutrients. Our advances in understanding the underlying molecular mechanisms of this protection already inform drug discovery programs of the most effective strategies to bypass the barriers. Further integration of biological, chemical and physico-mathematical approaches in addressing the remaining gaps in our knowledge is needed to obtain a sustainable pipeline of new antibacterials against Gram-negative pathogens.
References
- Rybenkov, V. V., Zgurskaya, H. I., Ganguly, C., Leus, I. V., Zhang, Z. & Moniruzzaman, M. (2021) The Whole Is Bigger than the Sum of Its Parts: Drug Transport in the Context of Two Membranes with Active Efflux. Chem Rev 121, 5597-5631.
- Acosta-Gutierrez, S., Ferrara, L., Pathania, M., Masi, M., Wang, J., Bodrenko, I., Zahn, M., Winterhalter, M., Stavenger, R. A., Pages, J. M., Naismith, J. H., van den Berg, B., Page, M. G. P. & Ceccarelli, M. (2018) Getting Drugs into Gram-Negative Bacteria: Rational Rules for Permeation through General Porins. ACS Infect Dis 4, 1487-1498.
- Cooper, C. J., Krishnamoorthy, G., Wolloscheck, D., Walker, J. K., Rybenkov, V. V., Parks, J. M. & Zgurskaya, H. I. (2018) Molecular Properties That Define the Activities of Antibiotics in Escherichia coli and Pseudomonas aeruginosa. ACS Infect Dis 4, 1223-1234.
- Richter, M. F., Drown, B. S., Riley, A. P., Garcia, A., Shirai, T., Svec, R. L. & Hergenrother, P. J. (2017) Predictive compound accumulation rules yield a broad-spectrum antibiotic. Nature 545, 299-304.
- Ude, J., Tripathi, V., Buyck, J. M., Soderholm, S., Cunrath, O., Fanous, J., Claudi, B., Egli, A., Schleberger, C., Hiller, S. & Bumann, D. (2021) Outer membrane permeability: Antimicrobials and diverse nutrients bypass porins in Pseudomonas aeruginosa. Proc Natl Acad Sci U S A 118.
- Isabella, V. M., Campbell, A. J., Manchester, J., Sylvester, M., Nayar, A. S., Ferguson, K. E., Tommasi, R. & Miller, A. A. (2015) Toward the rational design of carbapenem uptake in Pseudomonas aeruginosa. Chem Biol 22, 535-547.
- Durand-Reville, T. F., Miller, A. A., O’Donnell, J. P., Wu, X., Sylvester, M. A., Guler, S., Iyer, R., Shapiro, A. B., Carter, N. M., Velez-Vega, C., Moussa, S. H., McLeod, S. M., Chen, A., Tanudra, A. M., Zhang, J., Comita-Prevoir, J., Romero, J. A., Huynh, H., Ferguson, A. D., Horanyi, P. S., Mayclin, S. J., Heine, H. S., Drusano, G. L., Cummings, J. E., Slayden, R. A. & Tommasi, R. A. (2021) Rational design of a new antibiotic class for drug-resistant infections. Nature 597, 698-702.
- Leus, I. V., Adamiak, J., Chandar, B., Bonifay, V., Zhao, S., Walker, S. S., Squadroni, B., Balibar, C. J., Kinarivala, N., Standke, L. C., Voss, H. U., Tan, D. S., Rybenkov, V. V. & Zgurskaya, H. I. (2023) Functional Diversity of Gram-Negative Permeability Barriers Reflected in Antibacterial Activities and Intracellular Accumulation of Antibiotics. Antimicrob Agents Chemother, e0137722.
- Zahn, M., D’Agostino, T., Eren, E., Baslé, A., Ceccarelli, M. & van den Berg, B. (2015) Small-Molecule Transport by CarO, an Abundant Eight-Stranded β-Barrel Outer Membrane Protein from Acinetobacter baumannii. Journal of Molecular Biology 427, 2329-2339.
- Pelletier, M. R., Casella, L. G., Jones, J. W., Adams, M. D., Zurawski, D. V., Hazlett, K. R., Doi, Y. & Ernst, R. K. (2013) Unique structural modifications are present in the lipopolysaccharide from colistin-resistant strains of Acinetobacter baumannii. Antimicrob Agents Chemother 57, 4831-4840.
- Kim, S., Patel, D. S., Park, S., Slusky, J., Klauda, J. B., Widmalm, G. & Im, W. (2016) Bilayer Properties of Lipid A from Various Gram-Negative Bacteria. Biophys J 111, 1750-1760.
- Zahn, M., Bhamidimarri, S. P., Baslé, A., Winterhalter, M. & van den Berg, B. (2016) Structural Insights into Outer Membrane Permeability of Acinetobacter baumannii. Structure 24, 221-231.
- Abdul-Mutakabbir, J. C., Alosaimy, S., Morrisette, T., Kebriaei, R. & Rybak, M. J. (2020) Cefiderocol: A Novel Siderophore Cephalosporin against Multidrug-Resistant Gram-Negative Pathogens. Pharmacotherapy.
- Luna, B., Trebosc, V., Lee, B., Bakowski, M., Ulhaq, A., Yan, J., Lu, P., Cheng, J., Nielsen, T., Lim, J., Ketphan, W., Eoh, H., McNamara, C., Skandalis, N., She, R., Kemmer, C., Lociuro, S., Dale, G. E. & Spellberg, B. (2020) A nutrient-limited screen unmasks rifabutin hyperactivity for extensively drug-resistant Acinetobacter baumannii. Nature Microbiology 5, 1134-1143.
- Chan, D. C. K., Josts, I., Koteva, K., Wright, G. D., Tidow, H. & Burrows, L. L. (2023) Interactions of TonB-dependent transporter FoxA with siderophores and antibiotics that affect binding, uptake, and signal transduction. Proc Natl Acad Sci U S A 120, e2221253120.
- Szmelcman, S., Schwartz, M., Silhavy, T. J. & Boos, W. (1976) Maltose transport in Escherichia coli K12. A comparison of transport kinetics in wild-type and lambda-resistant mutants as measured by fluorescence quenching. European journal of biochemistry / FEBS 65, 13-19.
- Fukamizo, T., Kitaoku, Y. & Suginta, W. (2019) Periplasmic solute-binding proteins: Structure classification and chitooligosaccharide recognition. Int J Biol Macromol 128, 985-993.
- Tam, R. & Saier, M. H., Jr. (1993) Structural, functional, and evolutionary relationships among extracellular solute-binding receptors of bacteria. Microbiol Rev 57, 320-346.
- Higgins, C. F. & Gibson, M. M. (1986) Peptide transport in bacteria. Methods Enzymol 125, 365-377.
- Karwowski, J. P., Jackson, M., Theriault, R. J., Chen, R. H., Barlow, G. J. & Maus, M. L. (1989) Pacidamycins, a novel series of antibiotics with anti-Pseudomonas aeruginosa activity. I. Taxonomy of the producing organism and fermentation. J Antibiot (Tokyo) 42, 506-511.
- Mistry, A., Warren, M. S., Cusick, J. K., Karkhoff-Schweizer, R. R., Lomovskaya, O. & Schweizer, H. P. (2013) High-level pacidamycin resistance in Pseudomonas aeruginosa is mediated by an opp oligopeptide permease encoded by the opp-fabI operon. Antimicrob Agents Chemother 57, 5565-5571.
- Leimer, N., Wu, X., Imai, Y., Morrissette, M., Pitt, N., Favre-Godal, Q., Iinishi, A., Jain, S., Caboni, M., Leus, I. V., Bonifay, V., Niles, S., Bargabos, R., Ghiglieri, M., Corsetti, R., Krumpoch, M., Fox, G., Son, S., Klepacki, D., Polikanov, Y. S., Freliech, C. A., McCarthy, J. E., Edmondson, D. G., Norris, S. J., D’Onofrio, A., Hu, L. T., Zgurskaya, H. I. & Lewis, K. (2021) A selective antibiotic for Lyme disease. Cell 184, 5405-5418 e5416.
- de Matos, A. M. Recent Advances in the Development and Synthesis of Carbohydrate-Based Molecules with Promising Antibacterial Activity. (2023) European Journal of Organic Chemistry 26.
- Hancock, R. E. Aminoglycoside uptake and mode of action–with special reference to streptomycin and gentamicin. I. Antagonists and mutants. (1981) J Antimicrob Chemother 8, 249-276.
- Manioglu, S., Modaresi, S. M., Ritzmann, N., Thoma, J., Overall, S. A., Harms, A., Upert, G., Luther, A., Barnes, A. B., Obrecht, D., Müller, D. J. & Hiller, S. (2022) Antibiotic polymyxin arranges lipopolysaccharide into crystalline structures to solidify the bacterial membrane. Nat Commun 13, 6195.
- Ardebili, A., Izanloo, A. & Rastegar, M. (2023) Polymyxin combination therapy for multidrug-resistant, extensively-drug resistant, and difficult-to-treat drug-resistant gram-negative infections: is it superior to polymyxin monotherapy? Expert Rev Anti Infect Ther 21, 387-429.
- Geddes, E. J., Gugger, M. K., Garcia, A., Chavez, M. G., Lee, M. R., Perlmutter, S. J., Bieniossek, C., Guasch, L. & Hergenrother, P. J. (2023) Porin-independent accumulation in Pseudomonas enables antibiotic discovery. Nature 624, 145-153.
- Mao, W. M., Warren, M. S., Lee, A., Mistry, A. & Lomovskaya, O. (2001) MexXY-OprM efflux pump is required for antagonism of aminoglycosides by divalent cations in Pseudomonas aeruginosa. Antimicrobial Agents and Chemotherapy 45, 2001-2007.
- MacGowan, A. P., Attwood, M. L. G., Noel, A. R., Barber, R., Aron, Z., Opperman, T. J., Grimsey, E., Stone, J., Ricci, V. & Piddock, L. J. V. (2023) Exposure of Escherichia coli to antibiotic-efflux pump inhibitor combinations in a pharmacokinetic model: impact on bacterial clearance and drug resistance. J Antimicrob Chemother 78, 2869-2877.
- Lomovskaya, O. & Bostian, K. A. Practical applications and feasibility of efflux pump inhibitors in the clinic–a vision for applied use. (2006) Biochem Pharmacol 71, 910-918.
- Markham, P. N. Inhibition of the emergence of ciprofloxacin resistance in Streptococcus pneumoniae by the multidrug efflux inhibitor reserpine. (1999) Antimicrob Agents Chemother 43, 988-989.
- Lomovskaya, O. & Watkins, W. Inhibition of efflux pumps as a novel approach to combat drug resistance in bacteria. (2001) Journal of Molecular Microbiology and Biotechnology 3, 225-236.
- Oethinger, M., Kern, W. V., Jellen-Ritter, A. S., McMurry, L. M. & Levy, S. B. Ineffectiveness of topoisomerase mutations in mediating clinically significant fluoroquinolone resistance in Escherichia coli in the absence of the AcrAB efflux pump. (2000) Antimicrob Agents Chemother 44, 10-13.
- Gervasoni, S., Mehla, J., Bergen, C. R., Leus, I. V., Margiotta, E., Malloci, G., Bosin, A., Vargiu, A. V., Lomovskaya, O., Rybenkov, V. V., Ruggerone, P. & Zgurskaya, H. I. (2023) Molecular determinants of avoidance and inhibition of Pseudomonas aeruginosa MexB efflux pump. mBio, e0140323.
- Mehla, J., Malloci, G., Mansbach, R., Lopez, C. A., Tsivkovski, R., Haynes, K., Leus, I. V., Grindstaff, S. B., Cascella, R. H., D’Cunha, N., Herndon, L., Hengartner, N. W., Margiotta, E., Atzori, A., Vargiu, A. V., Manrique, P. D., Walker, J. K., Lomovskaya, O., Ruggerone, P., Gnanakaran, S., Rybenkov, V. V. & Zgurskaya, H. I. (2021) Predictive Rules of Efflux Inhibition and Avoidance in Pseudomonas aeruginosa. mBio 12.
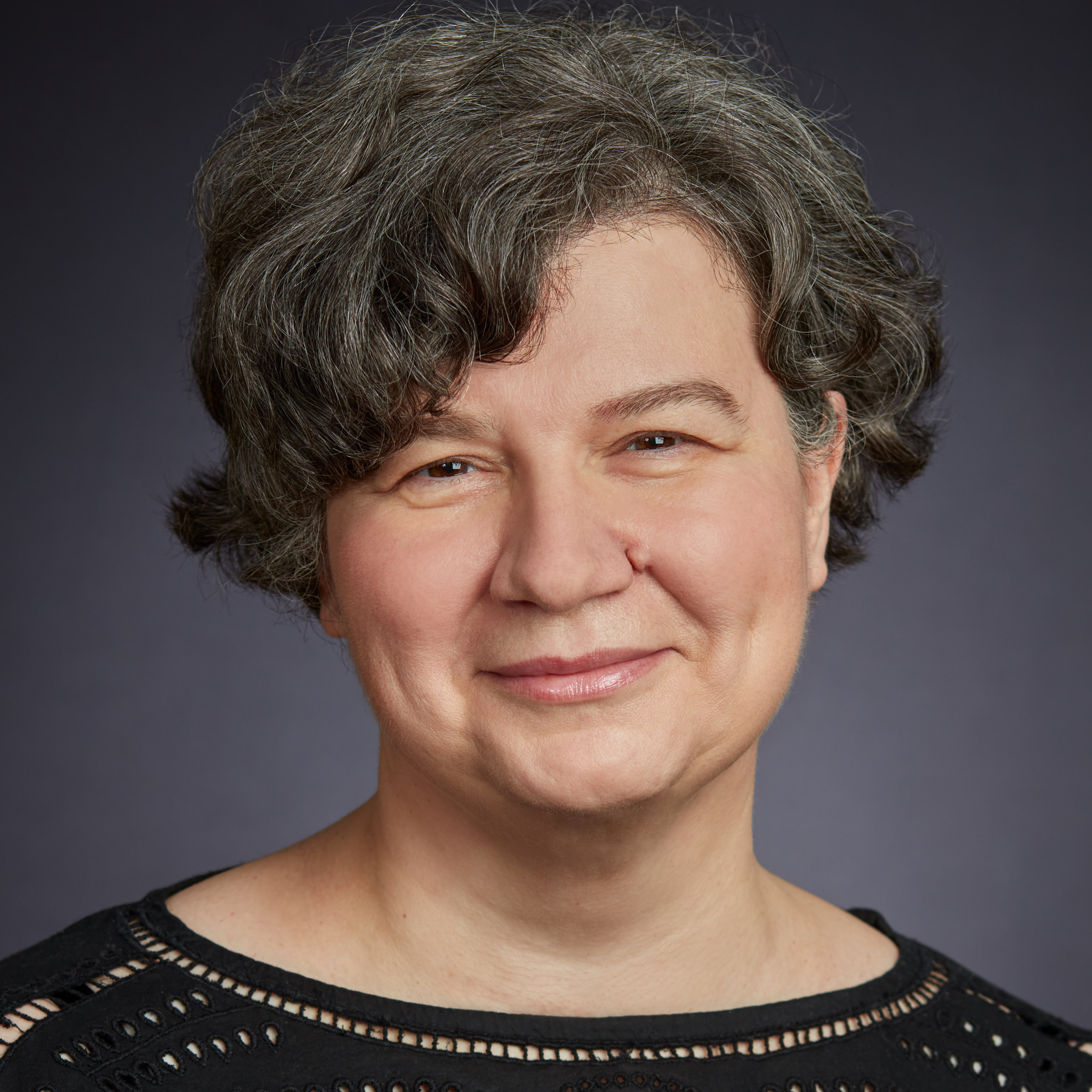
Helen I. Zgurskaya is currently George Lynn Cross Research Professor in the Department of Chemistry and Biochemistry at the University of Oklahoma, USA as well as a fellow of the American Academy for Microbiology and leads a research programme on antibiotic resistance, multidrug efflux mechanisms, and efflux pump inhibitors.
After receiving her MSc in microbiology from Dnepropetrovsk State University and her PhD in microbiology from the Russian Academy of Sciences, Helen completed postdoctoral fellowships at the Department of Microbiology and Immunology at Stanford University School of Medicine and the Department of Molecular and Cell Biology at University of California, Berkeley. She then joined the University of Oklahoma in 2000, where she went on to become an assistant professor and then a full professor in 2007.
Helen has written more than 60 papers and several book chapters. She has also helped prepare various conferences, been a member of different study sections at the National Institute of Health and reviewed proposals for the Wellcome Trust, the Medical Research Council and others. She is an associate editor of the ACS Infectious Diseases journal and a member of the editorial board for the journals; Antimicrobial Agents and Chemotherapy and Frontiers in Microbiology.
The author declares that she does not have any relationships or affiliations that could be construed as a potential conflict of interest.