2 June 2021
PLEASE NOTE: The Viewpoints on our website are to be read and freely shared by all. If they are republished, the following text should be used: “This Viewpoint was originally published on the REVIVE website revive.gardp.org, an activity of the Global Antibiotic Research & Development Partnership (GARDP).”
Due to the antibiotic resistance crisis that has dramatically emerged at the beginning of the new century, the past decade has seen a notable resurgence of clinical trials involving antibiotics1,2. Indeed, such a crisis prompted a remarkable effort in the discovery and development of new antibiotics active against the most problematic multidrug-resistant (MDR) pathogens such as carbapenem-resistant Enterobacterales (CRE), MDR Pseudomonas aeruginosa, carbapenem-resistant Acinetobacter baumannii (CRAb), vancomycin-resistant enterococci (VRE) and methicillin-resistant Staphylococcus aureus (MRSA), yielding a number of novel antibiotics that variably cover these resistant pathogens3,4. At the same time, several old antibiotics (e.g. fosfomycin and polymyxins) were repurposed as last resort agents against infections by some MDR pathogens (e.g. CRE and CRAb)5.
Under these circumstances, the conventional paradigm of clinical trials designed to demonstrate non-inferiority of new antibiotics vs. standard-of-care comparators within the context of a syndromic approach exhibited some limitations. This is because of the need to rapidly demonstrate the value of new or repurposed antibiotics against difficult-to-treat infections caused by MDR pathogens. Consequently, a “pathogen-specific” approach has increasingly been considered for the evaluation of new or old antibiotics for MDR infections as can be seen in various examples in the literature6,7,8,9.
Using a “pathogen-specific” approach
In the “pathogen-specific” approach, a major challenge is related to the patient enrolment step which, beyond the clinical syndrome, relies upon microbiological diagnosis of the causative agent in terms of bacterial species, resistance phenotype and/or mechanism. In fact, until recently, bacterial identification and antimicrobial susceptibility testing (AST) required a relatively long time (at least 2-3 days) due to reliance on conventional diagnostic tests based on bacterial culture.
When using RDTs based on molecular detection of resistance genes, one should be aware that the information obtained may be different from that provided as minimum inhibitory concentration (MIC) or zone diameters by phenotypic antibiogram obtained with a conventional dilution or diffusion test.
Recently, however, novel technologies that can significantly shorten the time-to-response (TTR) required for bacterial identification and AST have entered the diagnostic clinical microbiology practice. These technologies, henceforth referred to as rapid diagnostic tests (RDT), offer a major contribution to antimicrobial stewardship in clinical practice, but can also be useful to support clinical trials of antibiotics for MDR bacteria designed on a “pathogen-specific” approach.
Several RDTs are currently available for bacterial identification and AST, including:
- MALDI-ToF mass spectrometry platforms for rapid bacterial identification starting from isolated colonies or from positive blood cultures, which allow accurate bacterial identification in less than an hour and are also promising for rapid identification of some resistance mechanisms10,11.
- Molecular diagnostic platforms based on syndromic panels for the rapid genotypic identification of microbial pathogens and of clinically relevant drug-resistance determinants from isolated bacterial colonies, positive blood cultures or directly from some clinical specimens, with a TTR ranging from one to a few hours12.
- Lateral flow immunochromatography devices for rapid detection of some resistance mechanisms (e. g. carbapenemases, extended-spectrum-β-lactamases, VanA, PBP2a) from bacterial growth, with a TTR of 15-30 min13,14.
- Platforms based on advanced imaging technologies for rapid phenotypic AST, possibly in combination with in-situ hybridization for microbial identification, with a TTR of a few hours15.
Whole genome sequencing platforms, which can provide information about the presence of any pathogen in clinical specimens, could represent a further alternative in this area once these technologies allow the retrieval of information within a suitably short timeframe through a random-access approach16.
A case showing the usefulness of RDTs
A typical situation in which RDTs can be helpful in the diagnostic workflow is represented by bloodstream infections caused by Enterobacterales carrying carbapenemase genes (CPE). In this case, the rapid detection of a CPE in the blood culture by using syndromic panels based on real-time PCR/microarrays provides with valuable information about the nature of the infecting strain and its resistance mechanisms. For instance, the presence of a KPC-type carbapenemase suggests resistance to carbapenems and older β-lactams but susceptibility to novel β-lactamase inhibitor combinations (e. g. ceftazidime-avibactam, meropenem-vaborbactam and imipenem-relebactam) and to cefiderocol. On the other hand, the presence of a metallo-β-lactamase determinant (e. g. VIM or NDM) suggests resistance also to the new β-lactamase inhibitor combinations except those including aztreonam as a β-lactam agent.
When using RDTs based on molecular detection of resistance genes, one should be aware that the information obtained may be different from that provided as minimum inhibitory concentration (MIC) or zone diameters by phenotypic antibiogram obtained with a conventional dilution or diffusion test17,18,19. In fact, the results of molecular testing do not provide information about the susceptibility to antibiotics (e.g. MIC) of the infecting pathogens; it should be noted that the presence of a gene does not necessarily mean that it is expressed at a level conferring resistance as defined by the recommended clinical breakpoint concentrations. Moreover, the currently available syndromic panels only cover some clinically relevant drug-resistance mechanisms and will not detect those not targeted by the probes, including novel mechanisms that are not yet known20. Therefore, the molecular tests so far available can complement but not replace conventional phenotypic tests for antibiotic resistance profiling. In this perspective, RDTs that allow a rapid phenotypic AST (a few hours vs. 16-20 hours) can be coupled with molecular tests to shorten the overall diagnostic workflow.
Rapid diagnostic tests (RDT), offer a major contribution to antimicrobial stewardship in clinical practice, but can also be useful to support clinical trials of antibiotics for MDR bacteria designed on a “pathogen-specific” approach.
Obviously, the impact of RDTs may be dependent on a laboratory’s schedule and organisation. The highest throughput is obtained if the laboratory operates on a 24/7 schedule. Since the advantage of RDTs is reduced when diagnostic activities are limited to the daytime of working days. Finally, RDTs are more expensive than conventional technologies and require specific training for the lab personnel, although many technologies are quite easy to perform and require a relatively short hand-on time. Therefore, their implementation could be problematic or even impossible in lower-income settings.
In conclusion, RDTs are expected to help the clinical development of new antimicrobials by facilitating the rapid enrolment of patients in clinical trials designed with a “pathogen-specific” approach to evaluate new agents against specific categories of resistant pathogens (e. g. CRE, CRAb, VRE, MRSA).
References
- Boucher HW, Talbot GH, Bradley JS., Edwards JE, Gilbert D, Rice LB, Scheld M, Spellberg B., Bartlett (2009) Bad bugs, no drugs: no ESKAPE! An update from the Infectious Diseases Society of America. Journal of Clinical Infectious Diseases. 1:48(1):1-12.
- Rossolini GM, Arena F, Pecile P, Pollini S. (2014) Update on the antibiotic resistance crisis. Current Opinion in Pharmacology. 18:56-60.
- Abbas M, Paul M, Huttner A. (2017) New and improved? A review of novel antibiotics for Gram-positive bacteria. Clinical Microbiology and Infection. 23(10):697-703.
- Jean SS, Gould IM, Lee WS, Hsueh PR (2019) New Drugs for Multidrug-Resistant Gram-Negative Organisms: Time for Stewardship. International Society of Antimicrobial Chemotherapy (ISAC). Drugs. 79(7):705-714.
- Kaye KS, Gales AC, Dubourg G. (2017) Old antibiotics for multidrug-resistant pathogens: from in vitro activity to clinical outcomes. International Journal of Antimicrobial Agents. 49(5):542-548.
- McKinnell JA, Dwyer JP, Talbot GH, Connolly LE, Friedland I, Smith A, Jubb AM, Serio AW, Krause KM, Daikos GL; CARE Study Group (2019) Plazomicin for Infections Caused by Carbapenem-Resistant Enterobacteriaceae. New England Journal of Medicine. 380(8):791-793.
- Carmeli Y, Armstrong J, Laud PJ, Newell P, Stone G, Wardman A, Gasink LB. (2016) Ceftazidime-avibactam or best available therapy in patients with ceftazidime-resistant Enterobacteriaceae and Pseudomonas aeruginosa complicated urinary tract infections or complicated intra-abdominal infections (REPRISE): a randomised, pathogen-directed, phase 3 study. Lancet Infectious Diseases. 16(6):661-673.
- Bassetti M, Echols R, Matsunaga Y, Ariyasu M, Doi Y, Ferrer R, Lodise TP, Naas T, Niki Y, Paterson DL, Portsmouth S, Torre-Cisneros J, Toyoizumi K, Wunderink RG, Nagata TD. (2021) Efficacy and safety of cefiderocol or best available therapy for the treatment of serious infections caused by carbapenem-resistant Gram-negative bacteria (CREDIBLE-CR): a randomised, open-label, multicentre, pathogen-focused, descriptive, phase 3 trial. Lancet Infectious Diseases. 21(2):226-240.
- Motsch J, Murta de Oliveira C, Stus V, Köksal I, Lyulko O, Boucher HW et al. (2020) RESTORE-IMI 1: A Multicenter, Randomized, Double-blind Trial Comparing Efficacy and Safety of Imipenem/Relebactam vs Colistin Plus Imipenem in Patients With Imipenem-nonsusceptible Bacterial Infections. Clinical Infectious Diseases. 15:70(9):1799-1808.
- Jang KS, Kim YH. 2018 Rapid and robust MALDI-TOF MS techniques for microbial identification: a brief overview of their diverse applications. Journal of Microbiology. 56(4):209-216.
- Sauget M, Valot B, Bertrand X, Hocquet D. (2017) Can MALDI-TOF Mass Spectrometry Reasonably Type Bacteria? Trends in Microbiology. 25(6):447-455. doi: 10.1016/j.tim.2016.12.006.
- Couturier MR, Bard JD (2019) Direct-from-Specimen Pathogen Identification: Evolution of Syndromic Panels. Clinics in Laboratory Medicine. 39(3):433-451.
- Takissian J, Bonnin RA, Naas T, Dortet L. (2019) NG-Test Carba 5 for Rapid Detection of Carbapenemase-Producing Enterobacterales from Positive Blood Cultures. Antimicrobial Agents and Chemotherapy. 25:63(5):e00011-19.
- Matsui H, Hanaki H, Inoue M, Akama H, Nakae T, Sunakawa K, Omura S. (2011). Development of an immunochromatographic strip for simple detection of penicillin-binding protein 2′. Clinical and Vaccine Immunology. 18(2):248-53.
- Cenci E, Paggi R, Socio GV, Bozza S, Camilloni B, Pietrella D, Mencacci A. (2020). Accelerate Pheno blood culture detection system: a literature review. Future Microbiology. 15:1595-1605.
- Rossen JWA, Friedrich AW, Moran-Gilad J; ESCMID Study Group for Genomic and Molecular Diagnostics (ESGMD) (2018) Practical issues in implementing whole-genome-sequencing in routine diagnostic microbiology. Clinical Microbiology and Infection 24(4):355-360.
- The European Committee on Antimicrobial Susceptibility Testing. (2021) Homepage.
- Clinical and Laboratory Standards Institute (2018). Methods for dilution antimicrobial susceptibility tests. 13th Edition. CLSI standard M02.
- Clinical and Laboratory Standards Institute (2018). Performance standards for antimicrobial disk susceptibility tests for bacteria that grow aerobically. 11th Edition. CLSI standard M07.
- Arena F, Giani T, Pollini S, Viaggi B, Pecile P, Rossolini GM. 2017. Molecular antibiogram in diagnostic clinical microbiology: advantages and challenges. Future Microbiology. 12:361-364.
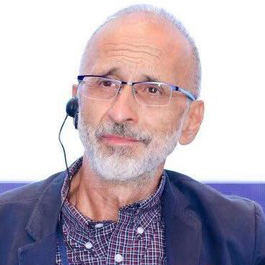
Gian Maria Rossolini is Professor of Microbiology and Clinical Microbiology at the University of Florence and Director of the Clinical Microbiology and Virology Unit at Florence Careggi University Hospital, both in Italy.
His current research interests mostly cover the fields of antimicrobial agents as well as antimicrobial resistance specifically its mechanisms, surveillance and molecular epidemiology. Gian Maria is also interested in antimicrobial susceptibility testing and novel diagnostic technologies in Clinical Microbiology. He has co-authored over 500 scientific papers and has 12 patent applications.
Gian Maria is an ESCMID fellow and has served on several committees of scientific societies or public institutions involved with antimicrobial agents and resistance. He has acted as a reviewer and advisor on behalf of several national and international funding agencies and Academic Institutions. He has served for ten years as an editor of Antimicrobial Agents and Chemotherapy and serves on the Editorial Board of several other scientific Journals.
The views and opinions expressed in this article are solely those of the original author(s) and do not necessarily represent those of GARDP, their donors and partners, or other collaborators and contributors. GARDP is not responsible for the content of external sites.