25 August 2022
PLEASE NOTE: The Viewpoints on our website are to be read and freely shared by all. If they are republished, the following text should be used: “This Viewpoint was originally published on the REVIVE website revive.gardp.org, an activity of the Global Antibiotic Research & Development Partnership (GARDP).”
Our antibiotic arsenal is becoming increasingly ineffective at treating bacterial infections. We are no longer discovering and developing new treatments fast enough to stay ahead of the growth in drug-resistant infections. These two simple and well-documented facts are at the crux of the antimicrobial resistance crisis1. The problem is multifaceted, with a complex combination of root causes requiring diverse strategies to deliver truly effective solutions.
A key component of these approaches is the continued development of new antibiotics and other direct-acting small molecule therapeutics – but how do we accomplish this? From a medicinal chemist’s perspective, there are many promising tactics. Note that the list below is not exhaustive and excludes other encouraging biological treatments such as phage therapy, antibodies, microbiome manipulation, immunomodulation and vaccines.
‘Traditional’ Antibiotics
New and Improved
The most successful tactic to generate new antibiotics over the past six decades has been to rationally improve the prevailing classes. This is why we have multiple generations of, for example, b-lactams, tetracyclines and quinolones. Medicinal chemistry optimization leads to new analogues with improved potency or pharmacological profiles, reduced side effects, or an ability to overcome resistance mechanisms. The advent of molecular design has aided the rational design of improved versions. For example, crystal structures and molecular modelling can be employed to identify the structural modifications in the ribosome caused by mutations conferring resistance to macrolides2 or oxazolidinones3, with new analogues designed to avoid steric clashes with the mutated residues. While there is a danger that this approach will lead to diminishing returns with only incremental improvements, the potential to create new subclasses with substantially better properties, such as the carbapenem b-lactam antibiotics, means that considerable effort is still expended. This is reflected by the predominance of drugs obtained via this approach in the clinical pipeline4.
The development of new methodologies has reinvigorated investigations of natural products as a source of novel antibiotics.
Rediscover old antibiotics
The ‘golden age’ of antibiotic discovery refers to the 1940s to 1960s, when scientists combed the world of natural products and uncovered completely new antibiotic classes. Because so many potential new antibiotics were being discovered, many were never advanced further or comprehensively examined. Now that our cupboard has become empty, it may be possible to find ‘diamonds in the rough’ by thoroughly searching the literature for interesting alternatives. This approach is exemplified by the ‘rediscovery’ of the octapeptins5, a lipopeptide class originally described in the 1970s but then largely ignored. However, given that these initial reports described that they retained activity against a current significant problem, polymyxin-resistant Gram-negative bacteria, they have now become the focus of a CARB-X (Combatting Antibiotic-Resistant Bacteria Biopharmaceutical Accelerator) funded medicinal chemistry optimization program.
Target-based approaches
Rational drug design based on interactions with specific protein targets has been highly successful for many therapeutic indications but not so much for antibiotic discovery. Bacteria are theoretically ideal organisms for this approach as they contain unique biological pathways and enzymes/receptors compared to their mammalian counterparts. However, summaries of research programs at GlaxoSmithKline6 and Astrazeneca7 demonstrate that, even though highly potent and selective compounds can be developed against an isolated target in vitro, whole-cell activity is usually absent as bacteria have evolved many mechanisms to prevent these molecules from reaching this target. Nonetheless, several programmes have persisted in reaching later development stages, such as inhibitors of LpxC8, a key enzyme in the synthesis of the membrane of Gram-negative bacteria. Whether these will lead to an approved antibiotic remains to be seen.
Natural product diversity
Almost all clinically useful antibiotics have been derived from natural sources, generally secondary metabolite products of soil-dwelling fungi or bacteria, particular actinomycetes9. The advent of modern medicinal chemistry relegated natural product discovery to a low priority, with most of the ‘low-hanging fruit’ already identified and screening campaigns only rediscovering known compounds. However, the development of new methodologies has reinvigorated investigations of natural products as a source of novel antibiotics.10 These include efforts to culture ‘hidden’ microorganisms found in soil which don’t grow using standard culture conditions or stimulating microorganisms to turn on defence mechanisms that produce ‘silent’ metabolites through co-culture or chemical treatment11. Genomic mining approaches identify biosynthetic gene clusters from individual microorganisms or environmental communities (metagenomics) and can be used to predict what types of novel structures they might produce12.
Synthetic chemical diversity
An alternative source of chemical diversity is synthetic chemical diversity. Chemists have been making compounds for various purposes for over 150 years, and most of these have never been tested for antimicrobial activity. Most drug discovery compound collections are now curated using ‘drug-like’ criteria, which exclude most existing antibiotic classes. A crowd-sourcing open-access initiative, the Community for Open Antimicrobial Drug Discovery (CO-ADD)13, is attempting to access unusual chemical diversity by providing free antimicrobial testing for chemists. Over 300,000 compounds have been assayed, with a significant number of ‘hits’. A recent analysis uncovered organometallic compounds as a promising new chemical class of antibiotics14.
Non-traditional antibiotics
In addition to developing traditional direct-acting small molecules, medicinal chemists have derived a range of less conventional therapeutic approaches. some of which are outlined below. These novel strategies are most often initially applied to cancer targets, with extension to antibacterial applications often lagging decades behind their initial discovery.
Antibody Drug Conjugates (ADCs)
A range of new oncology therapies has been developed by selectively targeting a cytotoxic agent to a cancer cell by linking it to an antibody that binds to specific motifs unique to the cancer cell. The same strategy can selectively target antibiotics to bacteria. However, there have only been a few examples to date, including a polymyxin analogue attached to a Gram-negative bacterial targeting antibody15, and a rifamycin analogue linked to an anti-S. aureus IgG1 monoclonal antibody, with the latter tested in a Phase 1 trial16.
We are no longer discovering and developing new treatments fast enough to stay ahead of the growth in drug-resistant infections.
Antibody-Recruiting Molecules (ARMs)
The ARMS approach17 highjacks the immune system to help destroy undesired cells. ARMs are bifunctional small molecules with one arm that binds to a cell surface biomarker and another acting as an antibody-binding terminus, using small molecules that elicit a non-specific antibody response. The resulting ternary complex is then disabled by antibody-dependent immune effector responses. While most often applied to cancer cells, attempts to target bacteria have also been described, such as using a DNA aptamer to bind to group A Streptococci (GAS), with an α-Gal epitope triggering the antibody response18.
Proteolysis-targeting chimera (PROTAC)
The PROTAC approach19, along with an ‘alphabet soup’ of acronyms of related approaches, hijacks the human proteolytic machinery to enzymatically degrade a protein of interest, e.g., rather than inhibiting an enzyme, the PROTAC approach destroys it. PROTAC therapeutics have reached Phase 2 clinical trials, but again with oncology indications. A recent review proposes how this strategy might be applied to bacteria;20 followed by a research report that describes a ‘BacPROTAC’ that hijacks a bacterial protease to degrade endogenous mycobacterium proteins21.
CRISPR-Cas-9
CRISPR-Cas9 therapeutics are ironically derived from a bacterial genome editing system. This highly precise gene-editing technology is used to modify, delete or correct specific regions of DNA. A clinical trial in 2021 proved that this approach could be used to delete a gene encoding a mutant protein in humans22. However, applications to kill bacteria are also possible and have been shown to work in vivo to kill S. aureus in a mouse skin colonization model23.
This quick overview of approaches to develop new antibacterial therapies shows that there are plenty of ideas ‘left in the tank’ and that as long as they are provided with sufficient time and money, the threat posed by antimicrobial resistance may not be as dire as feared.
References
- Cook, M. A.; Wright, G. D. The past, present, and future of antibiotics. Science Translational Medicine 2022, 14, eabo7793.
- Pavlova, A.; Parks, J. M.; Oyelere, A. K.; Gumbart, J.C., Toward the rational design of macrolide antibiotics to combat resistance. Chemical Biology and Drug Design. 2017, 90 (5), 641-652.
- Skripkin, E.; McConnell, T. S.; DeVito, J.; Lawrence, L.; Ippolito, J. A.; Duffy, E. M.; Sutcliffe, J.; Franceschi, F., Rχ-01, a New Family of Oxazolidinones That Overcome Ribosome-Based Linezolid Resistance. Antimicrobial Agents and Chemotherapy. 2008, 52 (10), 3550-3557.
- Butler, M.S. et al., Analysis of the Clinical Pipeline of Treatments for Drug-Resistant Bacterial Infections: Despite Progress, More Action Is Needed. Antimicrobial Agents and Chemotherapy. 2022, 66 (3) e01991-21.
- Velkov, T. et al., Structure, Function, and Biosynthetic Origin of Octapeptin Antibiotics Active against Extensively Drug-Resistant Gram-Negative Bacteria. Cell Chemical Biology. 2018, 25 (4), 380-391.
- Payne, D. J.; Gwynn, M. N.; Holmes, D. J.; Pompliano, D. L., Drugs for bad bugs: confronting the challenges of antibacterial discovery. Nat. Rev. Drug Discov. 2007, 6 (1), 29-40.
- Tommasi, R.; Brown, D. G.; Walkup, G. K.; Manchester, J. I.; Miller, A. A., ESKAPEing the labyrinth of antibacterial discovery. Nat. Rev. Drug Discov. 2015, 14 (8), 529-542.
- Mast, Y.; Stegmann, E., Actinomycetes: The Antibiotics Producers. Antibiotics 2019, 8 (3), 105.
- Moloney, M. G., Natural Products as a Source for Novel Antibiotics. Trends Pharmacol. Sci. 2016, 37 (8), 689-701.
- Ueda, K.; Beppu, T., Antibiotics in microbial coculture. The Journal of Antibiotics 2016, 70 (4), 361-365.
- Hover, B. M.; Kim, S.-H.; Katz, M.; Charlop-Powers, Z.; Owen, J. G.; Ternei, M. A.; Maniko, J.; Estrela, A. B.; Molina, H.; Park, S.; Perlin, D. S.; Brady, S. F., Culture-independent discovery of the malacidins as calcium-dependent antibiotics with activity against multidrug-resistant Gram-positive pathogens. Nature Microbiology 2018, 3 (4), 415-422.
- Frei, A., Zuegg J., Elliott A.G., Baker M, Braese S, Brown C., Metal complexes as a promising source for new antibiotics. Chemical Science. 2020, 11 (10), 2627-2639.
- Lovey, A. Krel M. Borchardt, A. Brady, T. Cole, J.N. Do, Q-Q. et al, Development of novel immunoprophylactic agents against multidrug-resistant Gram-negative bacterial infections. Antimicrobial Agents and Chemotherapy. 2021, 65, e00985-21.
- Peck, M. Rothenberg, ME, Deng R, Lewin-Koh N, She G, Kamath AV et al. A Phase 1, Randomized, Single-Ascending-Dose Study To Investigate the Safety, Tolerability, and Pharmacokinetics of DSTA4637S, an Anti-Staphylococcus aureus Thiomab Antibody-Antibiotic Conjugate, in Healthy Volunteers. Antimicrobial Agents and Chemotherapy. 2019, 63 (6), e02588-18.
- McEnaney P. J. Parker C.G. Zhang A.X. Spiegel D.A. Antibody-recruiting molecules: an emerging paradigm for engaging immune function in treating human disease. ACS Chemical Biology. 2012, 7 (7), 1139-51.
- Kristian, S.A. Hwang JH, Hall B, Leire E, Iacomini J, Old R, et al. Retargeting pre-existing human antibodies to a bacterial pathogen with an alpha-Gal conjugated aptamer. J Mol Med 2015, 93, 619–631.
- Békés, M.; Langley, D. R.; Crews, C. M. PROTAC targeted protein degraders: the past is prologue. Nature Rev. Drug. Disc. 2022, 21, 181-200.
- Powell, M.; Blaskovich, M. A. T.; Hansford, K. A. Targeted Protein Degradation: The New Frontier of Antimicrobial Discovery? ACS Infect. Dis. 2021, 7 (8), 2050-2067.
- Morreale, F.E. Kleine S, Leodolter J, 1, Junker S, Hoi DM,Ovchinnikov et al. BacPROTACs mediate targeted protein degradation in bacteria. Cell 2022, 185 (13), 2338-2353. DOI: 10.1016/j.cell.2022.05.009.
- Ledford, H. Landmark CRISPR trial shows promise against deadly disease. Nature 2021 Jun 29.
- Bikard, D. Euler CW, Jiang W, Nussenzweig PM, Goldberg GW, Duportet X et al.. Exploiting CRISPR-Cas nucleases to produce sequence-specific antimicrobials. Nat. Biotechnol. 2014, 32, 1146–1150.
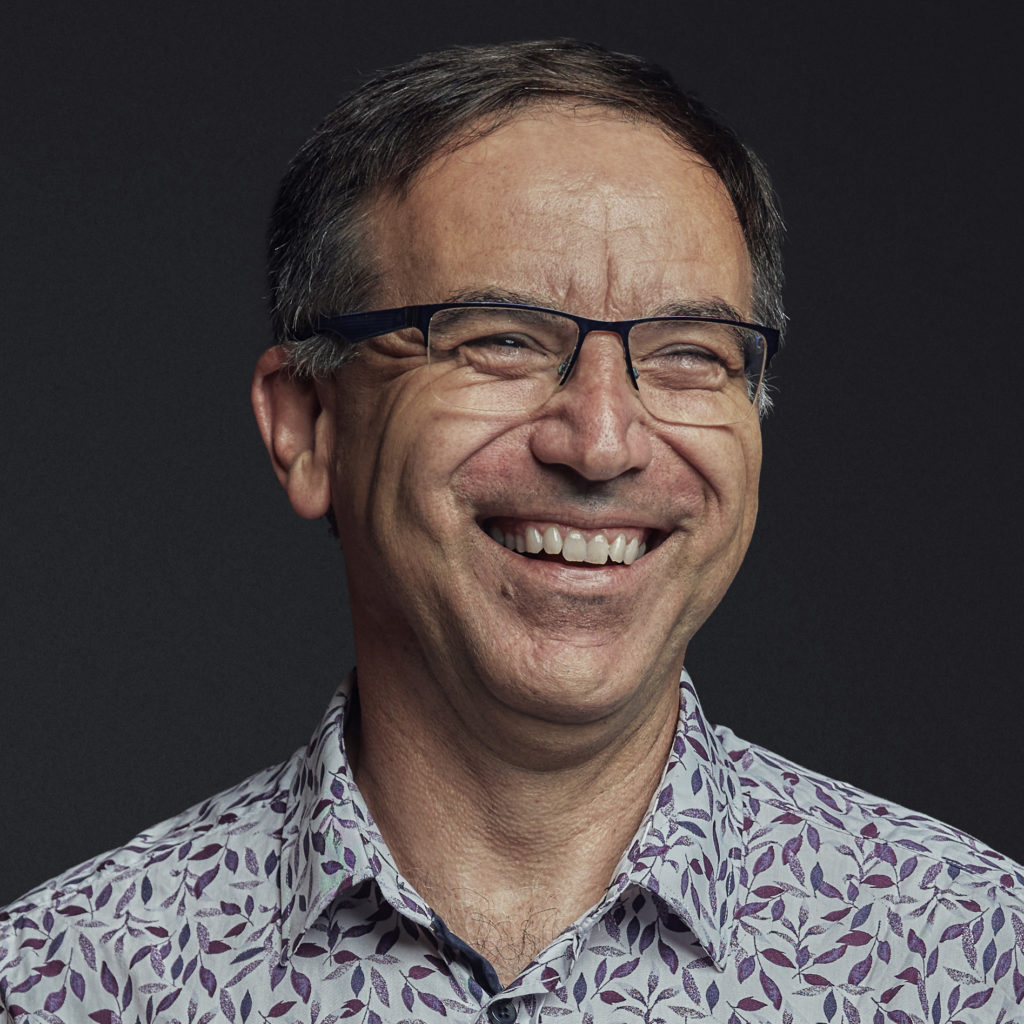
Mark A. T. Blaskovich is Director of the Centre for Superbug Solutions in the Institute for Molecular Bioscience at The University of Queensland, Australia. He is an ‘antibiotic hunter’ and medicinal chemist with 15 years of experience in industrial drug development. Before his academic career, Mark developed new antibiotics and non-antibiotic therapies to treat drug-resistant pathogens and also used modified antibiotics to detect bacterial infections.
He co-founded the Community for Open Antimicrobial Drug Discovery (CO-ADD), a global ‘crowdsourcing’ antibiotic discovery initiative, and has led industry collaborations focused on antibiotic development. Mark is an inventor on 11 patent families with over 50 granted patents. He has developed drugs in clinical trials, published over 120 research articles and secured over $20 million in antibiotic-related grant funding, including the first university-based award for antibiotic development from CARB-X (Combating Antibiotic-Resistant Bacteria Biopharmaceutical Accelerator). Mark is an Associate Editor of ACS Infectious Diseases.
The authors declare that they do not have any relationships or affiliations that could be construed as a potential conflict of interest.
The views and opinions expressed in this article are solely those of the original author(s) and do not necessarily represent those of GARDP, their donors and partners, or other collaborators and contributors. GARDP is not responsible for the content of external sites.